Synthesis of scenario results for all study sites |
![]() |
PESERA-DESMICE simulations were made for 12 study sites of the DESIRE study sites (see individual study sites sections for details). The DESMICE model was also applied in a non-spatially explicit manner to assess biogas as a desertification mitigation option in the Boteti area in Botswana (Perkins et al., in press) and is included in this cross-site analysis as well.
The remaining study sites have not been included in this report for a variety of reasons. In the Rendina basin (Italy) shallow landslides are the main land degradation problem for which PESERA was extended (PESERA-L ; Borselli et al, 2011). The temporal and spatial dimensions at which shallow landslides occur are not readily translatable in land use management options for which to conduct a cost-benefit analysis, and therefore the DESMICE model could not be applied. However, the results of PESERA-L are described in DESIRE report 82 (Borselli et al, 2011). The Nestos River Delta site (Greece) and two Russian study sites (Novy and Dzhanibek) feature salinization and water logging problems for which PESERA is not applicable. In principle, it would be possible to couple the DESMICE model with alternative models that are more suitable for these problems than PESERA. The biophysical model results for the Russian sites are presented in the individual study site sections.
PESERA Baseline runs
It is interesting to compare model assessment of soil erosion with land degradation mapping using expert knowledge (Figures 2 and 3). The latter was done in WB1 using the WOCAT mapping method (Van Lynden et al., 2011). When comparing Figure 2 with Figure 3 (taking care that not all sites feature in both charts), one can note:
The Tunisian site is the most arid, followed by the Spanish and Turkish sites, which overall seem to have more severe land degradation in expert opinion than model assessment. It could be that low levels of vegetation typical for those more arid conditions influence the experts, or that PESERA is too sensitive to slope angle in comparison to plant cover.
Technology scenarios
Table 1: Overview of technologies in each study site for which PESERA-DESMICE simulations were run and their classification according to main WOCAT categories: agronomic, management, structural & vegetative.
Next, we verified that for technologies modelled (under widely variable circumstances), most frequently about half of the study site can be treated due to applicability limitations. However, in some cases this is considerably less (checkdams for land – China: 9%; gully control by planting atriplex – Morocco: 10%) or more (terraces with pigeon peas – Cape Verde: 76%; rangeland resting – Tunisia: 69%). When aggregating per type of measures, management measures seem to have the widest range of applicability, followed by structural and agronomic measures (Figure 4B). It is suggested that vegetative measures typically demand more specific conditions and are consequently not as widely applicable.
Within applicable areas, many technologies are not profitable in about 70% of the area. Figure 4C shows aggregated financial feasibility of the technologies considered. This figure needs to be interpreted with caution as many factors come into play. For agronomic measures, effectiveness is an important factor. Yields may not respond or even be negatively affected, rendering the technology uneconomic despite low cost. For management measures, their versatile nature makes that although they are widely applicable, they are not universally financially sustainable. Together with structural measures, another factor with large influence is the time horizon after which the technology is evaluated. Some examples are included of measures that are not profitable after 10 years, but very profitable after 20 years. For structural measures, another factor that contributes to mixed financial performance is their sometimes very high investment cost. For the two vegetative measures, which are shown to be attractive in 100% of their applicability area, one should not forget that this is on a limited area – i.e. they may be highly specialized measures. More importantly however, the without case is unproductive in these cases, and the fact that plants need to grow to maturity means that the right time to evaluate the measure may be more easily determined.
Policy scenarios Comparing the per area unit costs of technologies with their effectiveness in reducing soil erosion, from a sample of policy scenarios for which cost data was available (n=5), a general trend of increasing effectiveness with increasing cost can be observed (Figure 5B). A much better correlation was found between total cost of a policy and its effectiveness in reducing soil erosion (Figure 8C). The difference between the two charts is that in the first instance, the area aspect relates to the cost of (subsidies towards implementation of) technologies on a per hectare basis, whereas in the second case the total cost of a policy can be high because of a large applicability area.
Global scenarios
Opportunities to reduce land degradation exist universally across applicability areas: at minimum, soil can be conserved by the technologies assessed on 70% of the applicable area. The rate by which soil loss can be reduced is either very high (80-100%) or moderate (0-40% reduction). In some cases, there are no additional costs involved to reduce soil loss, in others substantial investments (>€1000/ton) need to be made if analyses are done on a single year of erosion reduction. When spread out over the lifetime of technologies, erosion reduction becomes much more affordable, at rates often below €250/ton and in a considerable number of cases below €100/ton.
|
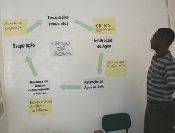
Acknowledgement
![]() |
The DESIRE project was
|
DESIRE brought together the expertise of
26 international research institutes
and non-governmental organisations.
This website does not necessarily
represent the opinion of the
European Commission. The European
Commission is not responsible for
any use that might be made of the
information contained herein.